High-temperature nuclear cycles
Introduction
Many high-temperature thermodynamic cycles are envisaged to produce electricity from nuclear reactors, as shown in this page of the portal.
We will limit ourselves here to presenting a Helium-nitrogen mixture Brayton cycle with intermediate exchanger and introducing supercritical CO2 cycles.
Pebble Bed Modular Reactor
PBMR (Pebble Bed Modular Reactor, Figure below) reactors are high temperature thermal neutron reactors with an output of about 100 MW using pebbles of weakly enriched uranium fuel embedded in carbon (which plays the role of moderator) and using helium as coolant.
Consider a helium Brayton cycle coupled to a nuclear reactor at 900 °C. In this cycle helium plays both the role of heat transfer fluid in the nuclear reactor, and of working fluid.
In the example shown in the figure below, a rate of 140 kg/s of helium is compressed to 70 bar (1), enters a regenerator, which it exits at 518 °C (2) before cooling the reactor core which it exits at 900 °C (3) and at 67.4 bar.
This flow is expanded in a HP turbine (3-5) used to drive the compressor, before entering a second turbine (5-6), which produces the useful power.
In Thermoptim, balancing a turbine with a compressor can be done by choosing the "mechanically balanced with" option located in the lower right part of the turbine screen (Figure below).
This option allows, for the "expansion" processes only, to determine the downstream pressure of the process such that the absolute value of the expansion work involved is equal to the work of the compression process chosen.
To choose the compression, just double-click in the field on the right of the check box, and choose from the list of proposed compression processes.
The cycle is closed by cooling the helium in two stages: regeneration (6-7) then cooling (7-8) to around 28 °C before it is compressed to 70 bar.
In this example, the polytropic efficiencies of the turbomachines are assumed to be 0.9, as well as the efficiency of the regenerator
Pebble Bed Modular Reactor with IHX
The simple helium Brayton cycle used for the PBMR reactor that we have just studied leads to excellent performance, but has the drawback that there are currently no suitable industrial turbomachines, and that it will take many years to develop them.
An alternative solution consists in using helium as coolant for the reactor core, and another gas as thermodynamic fluid, in particular a mixture of nitrogen and helium (79% N2, 21% He by volume), whose thermodynamic properties are relatively close to those of air for which industrial technology is well mastered.
This solution however requires the introduction of an intermediate heat exchanger called IHX for Intermediate Heat eXchanger (we then speak of an indirect Brayton cycle), the realization of which is not simple, even if it is more or less controlled, and which introduces irreversibilities which have the effect of lowering the overall efficiency.
Such a cycle is the subject of guided exploration (C-M4-V4).
Important note: to make this page easier for you to read, we have inserted here these guided explorations, but you should use the ones contained in the Thermoptim browser installed on your computer, because it is them that are coupled with Thermoptim and all the corresponding working files.
The figure below shows the synoptic view of such a cycle.
The drop in efficiency is significant. It is mainly due to pressure drops in the intermediate exchanger, which is a gas-gas exchanger whose heat exchange coefficients are low, which requires large exchange surfaces and small sections.
We assumed an average temperature difference of around 50 °C in this exchanger, but the price to pay is a consumption of more than 19 MW in the helium compressor and an overall loss of power of 32 MW.
MIT has recently worked on cycles using supercritical carbon dioxide, which it considers lead to better performance than others to produce electricity from nuclear reactors at moderate temperatures between 650 and 800 °C.
Proponents of these cycles argue that efficiencies are, for this temperature range, higher than those of steam cycles and that machines are much more compact.
Several types of supercritical CO2 cycles are considered: the simplest is a Brayton cycle with regenerator (Figure below) the principal variants involving partial cooling, pre-compression or recompression.
Simple regeneration cycle
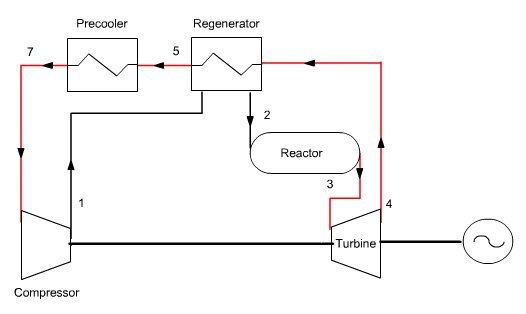
On the thermodynamics, the value of using such a cycle is to benefit from a compression work in supercritical liquid much lower than if the working fluid remains in the gaseous state as in a classic Brayton cycle.
In a 350-MWe supercritical CO2 simple regenerative cycle (Figure below), a flow of 3 t/s of CO2 at 200 bar enters the reactor at a temperature of 330 °C and leaves it at 650 °C. It is then expanded at the pressure of 77 bar in a turbine. Regeneration takes place between expanded CO2 and that which enters the reactor. CO2 then enters the cooler, which it leaves at 32.5 °C, before being sucked into the compressor, which brings it at 200 bar.
The efficiency of this cycle remains fairly low, close to 35% with a regenerator of effectiveness 0.9 and polytropic effi ciency turbomachinery equals to 0.9.
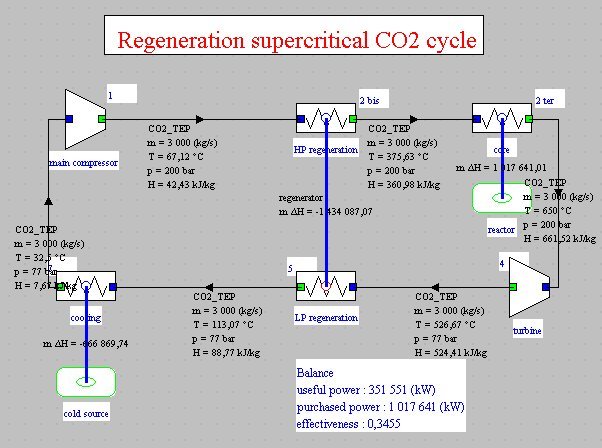
Simple regeneration cycle
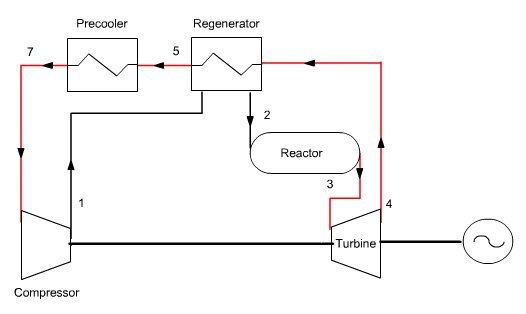
On the thermodynamics, the value of using such a cycle is to benefit from a compression work in supercritical liquid much lower than if the working fluid remains in the gaseous state as in a classic Brayton cycle.
In a 350-MWe supercritical CO2 simple regenerative cycle (Figure below), a flow of 3 t/s of CO2 at 200 bar enters the reactor at a temperature of 330 °C and leaves it at 650 °C. It is then expanded at the pressure of 77 bar in a turbine. Regeneration takes place between expanded CO2 and that which enters the reactor. CO2 then enters the cooler, which it leaves at 32.5 °C, before being sucked into the compressor, which brings it at 200 bar.
The efficiency of this cycle remains fairly low, close to 35% with a regenerator of effectiveness 0.9 and polytropic effi ciency turbomachinery equals to 0.9.
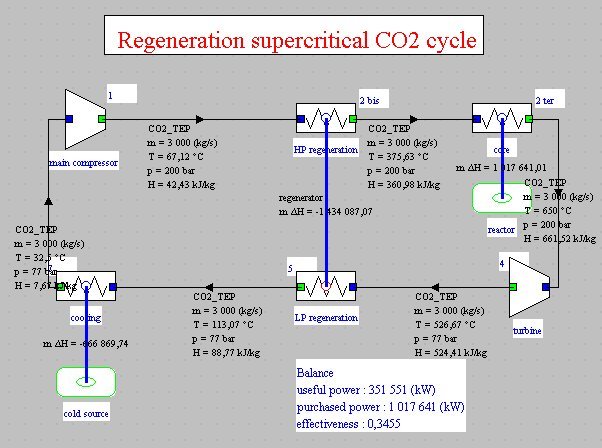
Recompression cycle
In a recompression cycle (Figure below), compression is two-stage with intermediate cooling of only a portion of the fluid, which allows recycling of a larger amount of heat.
The main flow of CO2 (3 t/s) leaving the turbine passes through a high temperature regenerator (4-5) and then in a low temperature one (5-6), before being split in two.
60% of the flow is cooled in a precooler (6-7), then compressed in the main compressor from 77 to 200 bar (7-8), and heated in the LT regenerator (8-9).
The remaining CO2 flow is compressed at 200 bar in the recompressor (6-8b), then mixed with another stream exiting the LT regenerator (1), and the total flow is heated in the HT regenerator (1-2) before entering the reactor.
CO2 exiting the reactor is then expanded in the turbine (3-4), and the cycle is thus closed.
In this case, the reactor inlet temperature is higher, and with the same assumptions as before on the polytropic efficiency of turbomachinery (0.9) and exchanger effectiveness (0.9), the capacity remains close to 300 MWe, and the efficiency exceeds 45% (Figure below). Even higher values are hoped for, the order of 50%, with exchanger effectiveness around 95%.
Supercritical CO2 cycles appear very interesting in thermodynamic terms. The two main technological constraints are the realization of regenerators in the circum-critical zone in particular to avoid any temperature crossing, and that of turbomachinery effective for CO2, an area where there is no reference. Moreover, these cycles can only work if the CO2 state at the precooler output is supercritical, which implies a temperature limit of 32 °C, which can be difficult to achieve when the cold source is outside air, a river or sea water.
Exercise
As an exercise, you can model the two cycles presented above. It is preferable that you have access to a thermodynamic property server such as CTP Lib to represent the thermodynamic properties of CO2). Its correction will provide the Thermoptim files allowing you to model the cycles presented above.